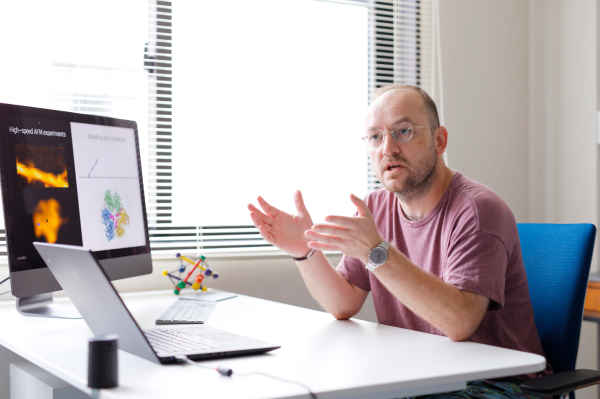
Holger Flechsig, Asst. Prof., Nano Life Science Institute (WPI-NanoLSI), Kanazawa University
“The goal of my research is to answer the central question of “How do proteins work”,” says Holger Flechsig, an expert in theoretical and computational biophysics with specific interest in molecular machines and motors, protein allostery, proteins interactions and cellular scale phenomena. “Before joining the Kanazawa NanoLSI in 2018 as an assistant professor in the Computational Science group, I focused on the functional dynamics of single proteins and it is known that specific functions of proteins are perfected by biological evolution and implemented by its conformational dynamics. I use multi-scale molecular dynamics simulations that enable us to produce molecular movies to visualize the dynamic motion of proteins.”
Some of the basic questions being addressed by Flechsig are:
- How does robust protein function emerge during biological evolution?
- What are the design and operation principles at the nanoscale?
- How do proteins interact and drive collective effects at the cellular scale?
“The answers to these questions are being applied to the interpretation and quantitative understanding of biological scanning probe measurements, including high-speed AFM,” says Flechsig. “Our computation can yield insights beyond the experimental limits in spatio-temporal resolution of AFM.”
Research methodology: Visualizing functional structural dynamics in proteins
Flechsig’s approach employs a variety of methods at different complexity levels. He is constructing mathematical models to understand protein operation using data from high-speed AFM measurements. Furthermore, Flechsig uses multiscale molecular dynamics simulations to visualize functional structural dynamics in proteins. “My approach allows the analysis of biomolecular dynamics with a spatio-temporal accuracy that is beyond the limitations of scanning probe microscopy experiments,” explains Flechsig. “It’s a powerful approach.”
Of major importance is the development of computational methods for quantitative analysis of biomolecular dynamics obtained from atomic force microscopy experiments. High-speed atomic force microscopy imaging only yields information of the surfaces of biomolecules and it is not possible to visualize atoms or individual amino acid residues. Therefore, not all functionally relevant structural dynamics is captured by such experiments.
“To overcome the limitations of HS-AFM, we have developed a unique computational framework to reconstruct 3D high-resolution atomistic structures from AFM topographic images obtained experimentally,” explains Flechsig. “We also work on analysis methods to advance quantitative assignment of molecular features from imaging. We have created the “BioAFMviewer interactive software suite” to make our developments available free to the scientific community.”
Recent findings: predicting 3D atomic structures from 2D AFM surface images
A recent high impact contribution includes computational mimicking of experimental scanning of biomolecular structures to compute pseudo-AFM images for comparison with experimental images. “Simulation AFM is the cornerstone method to predict 3D atomic structures from 2D surface scanning experiments,” stresses Flechsig. “For a given protein structure in all possible molecular orientations, simulation AFM can compute an endless library of simulation AFM images.”
In this method an algorithm detects and picks an individual simulated image that best matches a target image from an experiment. Then, automatized fitting of available high-resolution protein structures allows to infer 3D atomistic conformations from surface imaging, and, in principle an atomistic-level understanding of resolution-limited measured images becomes possible. “Using this method we constructed full atomistic models of protein lattices from measurements, or we were able to assign catalytic states to measured enzyme shapes which allows detailed kinetic analysis,” says Flechsig.
Open science, imaging “icebergs”, and the interactive BioAFMviewer software suite
Flechsig and colleagues have developed the BioAFMviewer software package to freely-share their expertise in simulating AFM images and automatized fitting of high-resolution structural data into experimental AFM images with the global scientific community.
“This software provides the platform to employ the enormous volume of high-resolution structural and modelling data to facilitate interpretation of resolution-limited AFM images,” says Flechsig. “We can illustrate the advancements made with reference to an iceberg. Our BioAFMviewer enables you to see not just the tip of an iceberg but also everything hidden under the sea, to the extent that you can even detect impurities or density differences within its structure, thereby explaining for example the coloration of icebergs. In the same way simulation AFM and automatized fitting now allows us to extract 3D atomistic information from experimental imaging of biomolecular surfaces.” The BioAFMviewer software has a wide range of applications and is important for many projects at NanoLSI. “I am proud to say that the BioAFMviewer software has become a standard tool used by the Bio-AFM community worldwide,” adds Flechsig. “And there have already been dozens of applications in published work.”
Future Plans
Flechsig says that he and his colleagues will continue to improve the interpretation of scanning probe measurements including advanced computational methods that combine molecular modelling and image analysis to overcome the experimental resolution limits.
“Eventually, the aim is to reconstruct a 3D high-resolution molecular movie with atomistic information from a HS-AFM topographic movie,” says Flechsig. “This is really challenging. I also want to address other fundamental questions in the field of physics of living matter and expand my research towards understanding biological phenomena at the cellular level.
Research highlights
In a recent article, Flechsig and colleagues describe the mathematical methods and computational framework for automized fitting of atomic biomolecular structures into experimental images [1]. The applications range from single molecular machines, protein filaments, to large-scale protein lattices. These results demonstrate how 3D atomistic information obtained from resolution-limited AFM topographic images advances the molecular understanding beyond measurements.
Details about the freely available BioAFMviewer software platform for simulated AFM scanning of protein PDB data were reported in 2020 [2]. This software allows the use of enormous amounts of high-resolution biomolecular structures and modelling data to facilitate the interpretation of AFM imaging. The BioAFMviewer has become a standard tool used by the Bio-AFM community worldwide.
- Amyot R, Marchesi A, Franz CM, Casuso I, Flechsig H. Simulation atomic force microscopy for atomic reconstruction of biomolecular structures from resolution-limited experimental images. PLoS Comput. Biol. 18(3): e1009970 (2022).
- Amyot R, Flechsig H. BioAFMviewer: an interactive interface for simulated AFM scanning of biomolecular structures and dynamics. PLoS Comput. Biol. 16(11): e1008444 (2020).
Further Information
Asst. Prof. Holger Flechsig’s episode on the NanoLSI Podcast.
Posted Mar. 13, 2023